Electron microscopy: Structure from pictures
by Helen Saibil, Birkbeck College, London
Transmission electron microscopy (TEM) is a very
direct way of determining macromolecular structure, which has reached near-atomic
resolution for protein structures in the 1990s. In the 1930s, Ruska built the
first EM, using electromagnetic lenses to focus the electron beam. The electron
gun, lens and camera systems were enclosed in an evacuated column, into which
the thin specimen was introduced. Because the electrons scattered from the specimen
are recombined by the objective lens to form a magnified image, the phase information
is retained. Thus, no additional experiments are necessary to obtain phase information
as is the case in X-ray crystallography.
The electron wavelength is ~0.05 angstrom, but limitations on electron lens
quality necessitate the use of small apertures that restrict the microscope
resolution to ~1 angstrom. This resolution can be obtained with modern TEMs
from thin specimens that scatter strongly and are insensitive to electron beam
damage.
3D structure from 2D projections.
The requirement for a small objective lens aperture has an important consequence
for structure determination by TEM of thin specimens. The restriction on
scattering angle by the aperture results in a large depth of focus. This
means that the whole specimen is imaged at the same focus, and the recorded
image is a 2-dimensional projection of the 3D electron scattering density
of the specimen. It is therefore possible to reconstruct the 3D structure
by recording projections of the object from different view directions.
If the relative angles of the views can be determined, the 3D structure
can be reconstructed by tomography.
Imaging biological structures.
What about biological specimens, that are made of weakly scattering, beam-sensitive
molecules? In the 1950s, the membrane topology of cellular structures such as
the mitochondrion was first imaged in thin sections of fixed, dehydrated and embedded
biological tissue. The sections were stained with heavy metal salts to make the
protein and lipid structures visible. In tissue sections, the fine details (below
~50 angstrom) of the biological structure are lost during the preparation procedure.
Moving towards virus and macromolecular structure.
In the 1960s, negative staining came into use. This is a simple and extremely
effective way to view the overall shape and symmetry of macromolecular
complexes. A solution of the complex is dried down in a layer of heavy
metal stain on a support film. The surface shape of the complex is visible
in negative contrast, to a resolution of ~20 Å. 3D reconstruction
is possible, but the drying step tends to flatten the structure onto the
support plane, causing distortions to the 3D structure. Although it is
the stain envelope and not the native structure that is being imaged, negative
staining is often very useful and informative.
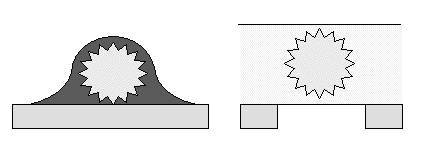 |
Schematic edge-on views of
EM grids of a virus, negatively stained (left) or trapped in a layer of
vitreous ice for cryo EM (right).
Image processing
Beginning in the 1960s, and through the 70s and 80s the major image processing
methods for biological structures were established, for symmetry analysis
and 3D reconstruction of icosahedral viruses, helical fibres and 2D crystals.
A somewhat different set of methods was developed for single particles
(isolated macromolecular complexes of any symmetry).
Cryo EM
The major development of the 1980s was the invention of cryo EM. This largely
solved 2 major problems that had held up the field: damage to the specimen
by electron irradiation and by the dehydration necessary for stability
in the high vacuum environment inside the microscope. An EM grid with a
thin layer of solution containing the macromolecular structure is trapped
in a solid, glass-like state vitrified water by plunging into liquid ethane.
The grid is transferred and maintained in the microscope with liquid nitrogen
cooling. The low temperature stabilizes the vitrified sample in the vacuum,
and moreover slows down the rate of electron beam damage. Thus, the native
hydrated structure is observed directly, although with extremely low contrast
and signal:noise.
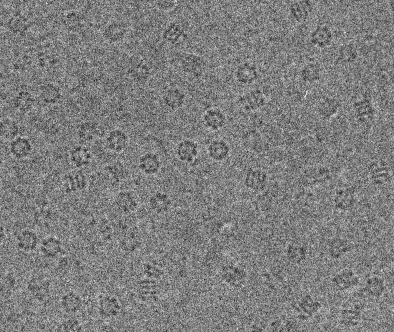 |
Cryo EM image of GroEL chaperonin
complexes, showing end views (rings) and side views (stripes).
Structure determination
In the 1990s, cryo TEM has developed into a major tool for structural biology.
Near atomic resolution has been achieved for 2D crystals of the membrane
proteins bacteriorhodopsin and plant light harvesting complex, and also
for tubulin. Sophisticated disorder correction methods have been developed
for images of 2D crystals and helical assemblies. The most rapid development
has been the increase in resolution obtainable with single particles (~7
Å for hepatitis B core particles), resolving alpha-helical secondary
structure. This advance is due to improvements in high resolution contrast
of weakly scattering specimens, obtained by using field emission gun microscopes,
improvements in computer processing and the use of much larger data sets.
General single particle software has been developed for the most difficult
type of sample - the ribosome, an asymmetric single particle. One of the
major challenges facing the field is the atomic structure determination
of such a sample. It seems likely that this will occur in conjunction with
X-ray crystallography studies.
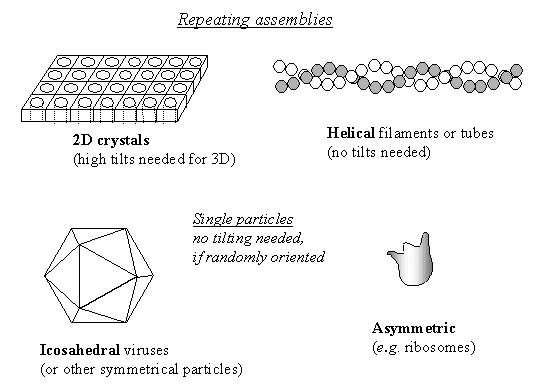 |
Sample geometry for structure determination
by cryo EM and 3D reconstruction.
Combination of EM with X-ray or NMR data
A very productive approach to understanding the structure of large complexes
or those with mobile components in different states is to fit components of
known atomic structure as rigid bodies into EM maps of larger complexes. Conversely,
EM density maps can be used to provide a starting model for phasing X-ray crystallographic
data.
Example: Large ribosomal subunit
Time-resolved cryo EM
Because of the speed of the vitrification step, it is possible to spray
a reagent onto the EM grid just before it plunges into the ethane, and
trap a reaction product within a few milliseconds. This method can thus
provide structures of very short lived transient states.
[BACK]